QuesTek’s ICMD: Faster, cheaper, and better alloy development for Additive Manufacturing
Alloy development has evolved dramatically throughout history, from what was a game of 'trial and error' to a systematic approach driven by Design of Experiments and specialist software. Now, the adoption of Integrated Computational Materials Engineering (ICME) is once again changing the way new materials are developed and deployed in today's advanced manufacturing technologies. QuesTek's Keith Fritz, Director of Solutions Architecture, details how the company's ICMD® platform is enabling faster, cheaper and more successful development of new alloys for metal Additive Manufacturing, as well as the build parameters to process them. [First published in Metal AM Vol. 8 No. 4, Winter 2022 | 15 minute read | View on Issuu | Download PDF]
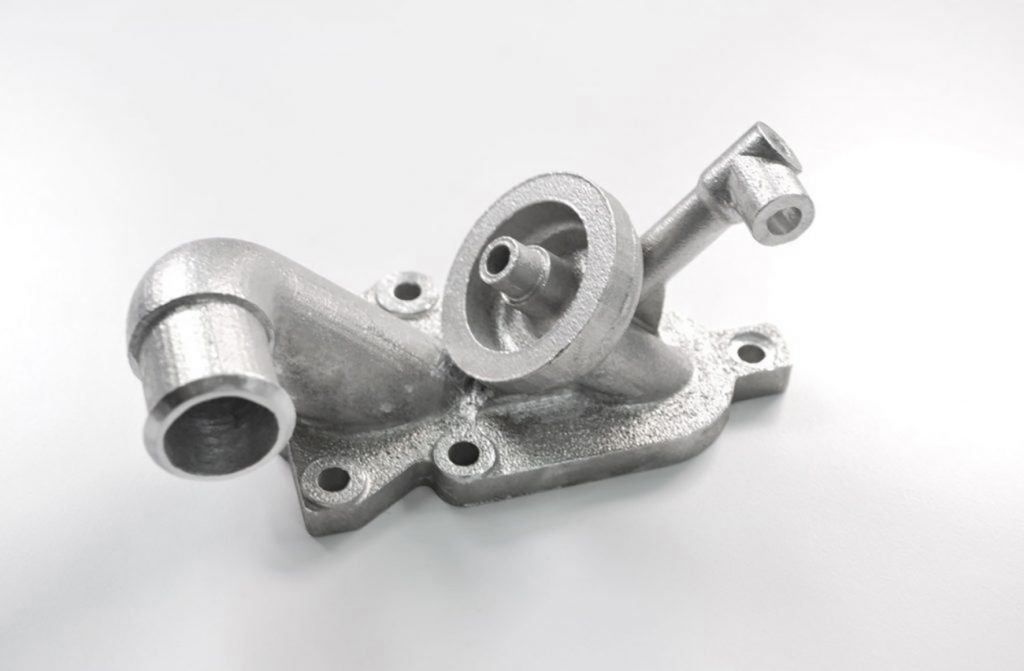
The way materials engineers develop novel alloys has changed radically over time. In early foundry work, alloy development was a game of trial and error. At the direction of the person most familiar with the practice, materials engineers would make minor changes to the material composition and/or process, then capture and measure the results downstream. The results of these trials could be a little fuzzy; it was not always clear how impactful the ‘change’ was, and what was really driving the results.
The next substantial development was the widespread adoption of lean six sigma, in particular the Design of Experiment (DoE) approach combined with the statistical software Minitab®. This democratised experiments and gave engineers access to tools that enabled them to approach alloy development systematically. Six sigma provided a structure for experiments and a clear ‘line in the sand’ – decided by statistics – that determined the most impactful factors.
A major shift in manufacturing was the introduction of Additive Manufacturing. The reality of building three-dimensional metal objects which could never have been manufactured by conventional means was a radical departure from subtractive-minded designs and tooling-heavy processes.
This brings us to today, where the adoption of Integrated Computational Materials Engineering (ICME) promises to change the way new materials are developed and deployed. Through the creation of its unique ICMD® software platform, QuesTek hopes to enable each and every materials engineer to accelerate the development and adoption of new alloys for AM.
QuesTek aims to empower innovators by resolving material challenges. The company is currently a market leader in Integrated Computational Materials Engineering; our Materials by Design® technologies have been proven to reduce the development time and cost, and increase the performance, of novel materials. We are the only company in its market space that can take an innovative design through production, certification, and flight qualification using its own materials, in a fraction of the time and cost of traditional, or purely algorithmic, methods.
What is ICMD?
Integrated Computational Materials Design combines fundamental computational physics and informatics with systematic experimentation and advanced manufacturing. It integrates a top-down methodology, using informatics tools to mine materials databases, product characteristics, and manufacturing process performance with bottom-up multiscale modelling based on fundamental physics. ICMD places a major emphasis on the process-structure-properties-performance (PSPP) relationship to gain an understanding of how processes produce material structures, how those structures give rise to material properties, and how those properties produce specific performance, to enable the better selection and development of materials for a given application. Both process-structure and structure-property models are examined and modified in ICMD to optimise results. It is the ‘I’ (Integrated) in ICMD that counts; the integration of multiple materials modelling tools linked together is what supports a full understanding of selection, development, and performance in an Additive Manufacturing application.
In this model of a material, the four components are linked with physics-based models of the interactions. The model starts with the process. In the case of Additive Manufacturing, the process includes the elemental composition of the alloy, the powder characteristics, thermal histories, and various laser parameters. By inputting this process, the microstructure of the material can be predicted using first principle, fundamental physics. Such structures include matrix phase properties, grain structure, solidification characteristics, defects that may be generated, and more. Once the structure of a material has been predicted, another set of physics-based models is used to predict the properties associated with that structure. Typical material properties include density, mechanical properties, electrical conductivity, and susceptibility to hot cracking, to name a few. Once the properties of the material are determined, yet another set of physics-based relationships can be applied to the material’s performance. This enables the user to target a broad spectrum of performance attributes, from toughness, thermal properties, fatigue, corrosion, and creep, to sustainability.
How ICMD is applied to Additive Manufacturing process parameter sets
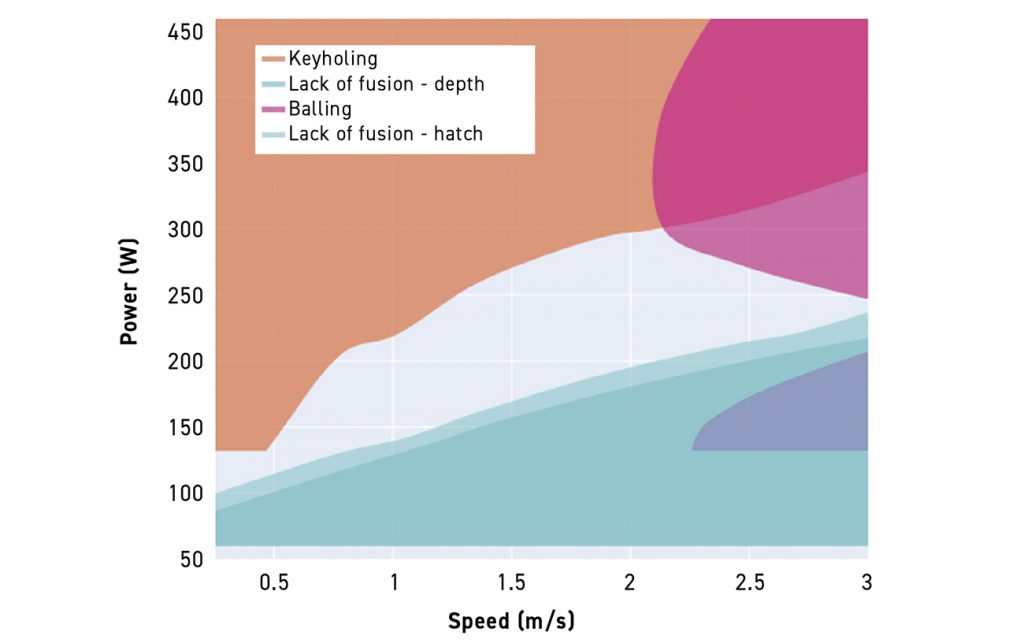
In Additive Manufacturing processes, to model melt pool dynamics, QuesTek creates a testing matrix of various laser speeds, power, and hatch distances. Once the samples are made (specific to the machine and alloy), we measure the melt pool characteristics, including width and penetration depths. With that, we can predict the optimal machine parameters. We can model weld balling, keyholing, and lack of fusion defects for both hatch and depth. Using those process-calibrated models, we generate a processing map to identify the optimal parameter set in the tradeoff between throughput and part quality. Fig. 2 shows an example of an ICMD-produced additive manufacturability map. The area in grey would be considered the optimal processing range.
Understanding how this map is created provides processing guidance in both directions. Once a process is established, one can look at a raw material input change (let’s say a new material lot with different chemistry) and determine how that new material chemistry might respond at the established parameters, without even a sample build. Fig. 3 shows how changes in elemental composition would impact material properties This is a great tool for overall quality control and incoming raw material disposition.
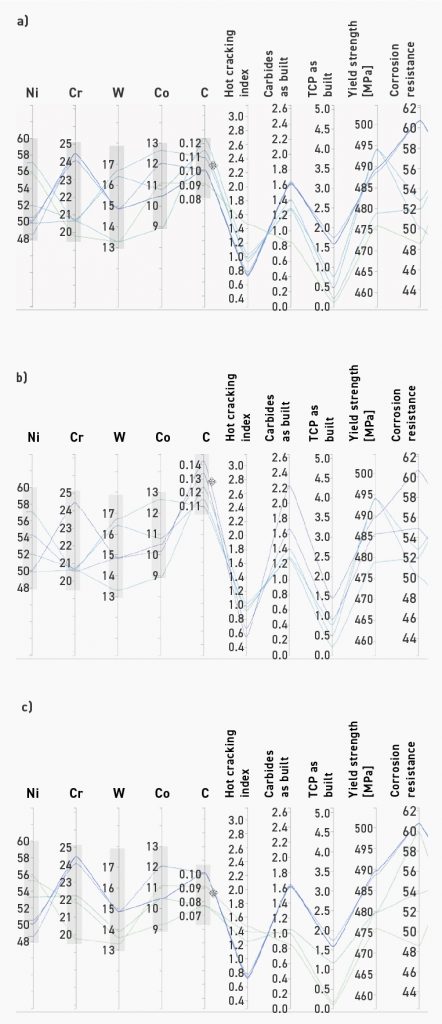
Development of a parameter set with a traditional DoE approach could take well over a year to complete, focusing only on density and keyhole defects. This ICMD approach takes only several weeks to a few months, and creates data and models that can later be used to produce answers to more complex questions such as fatigue life, making it uniquely suited to address industry challenges in a fraction of the time.
Expedited development of AM parameters for new alloys
One of the most important opportunities to take advantage of ICMD is the development of robust AM regimes for new alloys. It becomes exceptionally easy to query material compositions as they relate to process prediction and subsequent material structure. Fig. 3 shows an example of an interactive chart of elemental composition and properties that a designer can manipulate to query various compositions and how changes affect properties.
Using ICMD, a designer can quickly vary the composition of any of the alloying elements and see how those changes affect critical build parameters as well as desired properties. As a demonstration, Fig. 3 shows only a portion of the alloy and a portion of the properties; the chart is far more comprehensive in practice.
QuesTek’s background in Additive Manufacturing
QuesTek has a long history in Additive Manufacturing, having completed over seventy different engagements. Clients have included the US Defense Advanced Research Projects Agency (DARPA); the US Army, Navy, and Air Force, and numerous corporate entities. Materials addressed by the engagements include nickel superalloys, aluminium, titanium, stainless steels, niobium, tungsten, magnesium, high-entropy alloys, Invar, metallic glasses, and other complex alloy systems. QuesTek deliverables in AM have been consistently recognised by leading materials forums.
For example, in 2020, QuesTek received first place in the America Makes-Air Force Research Lab AM Modeling Challenge Series, by delivering improved understanding of the structures and performance of additively manufactured metallic components made from IN625 superalloy. In 2022, QuesTek won first place for Best Modeling Results Predicting Macroscale Tensile Behavior of IN625 from The AM Best Awards, a joint programme of the National Science Foundation (NSF) and the Minerals, Metals, and Material Society (TMS).
Digital twin and modelling
QuesTek’s models are not only created in cyberspace. Models are calibrated and validated using samples produced by the same operation that is being modelled. This practice is what gives our models their predictive power and trusted outputs. After the process is modelled, calibrated, and validated, you have created a digital twin of the process. This digital twin of the additive process has unique benefits that give businesses an edge in the market.
In one scenario, modelling allows us to predict the best yield strength as a function of alloy composition and processing conditions. This helps us understand which energy densities might be optimised within a given set of AM bounds. Fig. 4 shows the light blue lines superimposed on the Solidus mass fraction plot. These are expected yield strength curves as a function of solidus temperature versus aluminium mass fraction in titanium base metal.
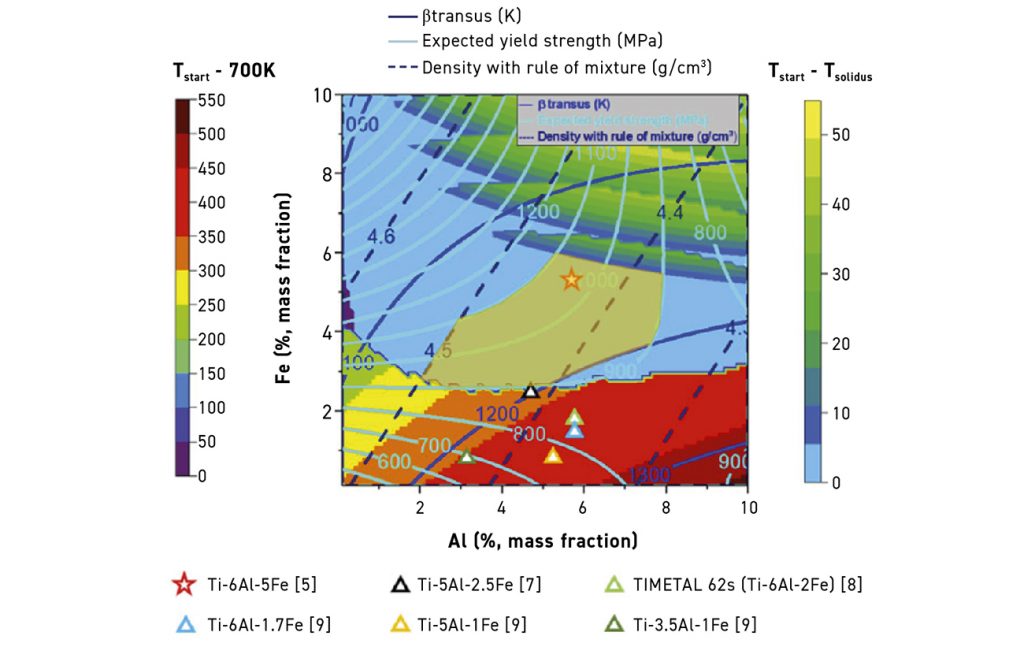
QuesTek’s ICMD approach has other strong advantages beyond faster development time. For part qualification, ICMD can reduce the number of tests needed. Instead of having to produce and test samples over the entire design space – which can be extremely lengthy and costly – ICMD can drastically lower the number of tests required by targeting only high informational value samples. Recently, QuesTek formulated an approach to accelerate qualification for fatigue critical materials, wherein digital twins of fatigue samples are generated to simulate fatigue tests, reducing the testing needed to establish a material dataset for one client by 60%.
This approach provides three key benefits in the qualification of additively manufactured materials: (1) reduced cost, (2) reduced time, (3) increased mechanistic understanding of the key features driving fatigue. Though ICMD is not a surrogate for required testing for AMS or MMPDS qualification, it plays a significant role in carefully expediting part of the design, development, and qualification process. Being at the centre of digital transformation alongside machine learning, we may think of the day when ICMD (and other computational tools) will be used in place of the required tests for these standards organisations, but do not get too nervous – this is a long way from where we are today.
That is not to say that ICMD is not valuable in MMPDS qualification. On the contrary, it can be critical in many instances. Here is a quick story for you: Prior to engaging with us on ICMD, a (now) client of ours had intended to use a custom heat treatment cycle for their additive alloy, but when they went to their OEM partners, the cost of this custom cycle was prohibitive, it was more expensive than the standard cycles the partners had budgeted for. The company moved ahead, making qualification samples with a less expensive standard heat treatment cycle. The mechanical testing for these samples did not perform as expected, with the standard deviation showing 2–3 X higher than anticipated.
When engaged to perform a post-mortem investigation, it was revealed that the heat treatment was too close to the formation of a deleterious phase. QuesTek’s ICMD digital twin of the process would have seen this issue before considerable resources were used in producing and testing samples. If the standard heat treatment was a fixed constraint, it may have been possible to make some adjustments to the alloy chemistry or build parameters to avoid this deleterious phase region. In this way, ICMD can act as an insurance policy or final check before hundreds of thousands of dollars and months of time are spent testing samples. Once the ICMD models are made and calibrated, you can be sure of the results of physical testing within narrow statistical bounds.
Process optimisation
Once these models, or digital twins, of the process are produced and calibrated, they are not specific to one area. Models are not generated separately for the process parameters, fatigue, or heat treatment simulation, but are linked together to represent the entire process. This digital twin approach allows you to optimise the process and investigate how different variables may impact the process without having to change machine settings or produce custom alloy formulations.
In most operations, there is a nagging question about the pickup of trace elements from the reuse of powder or remelting of scrap parts back into master heats. Having the ICMD digital twin, we can simulate what an increase of 10–100 ppm of nitrogen would impact to the microstructure and, thus, the final performance of the parts. This can help put to rest sometimes decade-long arguments, and it also may show the process has some robustness that would allow for increased scrap use or more reuse cycles for a lot of powder.
Materials concurrency
The concept of material concurrency states that the material can be engineered in parallel with the product it is to be used for, and, thus, the material can be optimised for its application. Often, in the AM space, the materials being used are not given proper consideration. In the early days of AM, common and well-known alloys would be turned into powder for AM. It was a hard lesson to learn that many of these alloys were not optimal for Additive Manufacturing. It was not until later that alloys custom tuned to the challenges of AM would come along, and QuesTek’s patented alloys have been optimised to produce desirable properties unique to the AM process. Materials concurrency is the design of the material to mate with the process to create an optimised product. Novel materials enable novel products.
QuesTek’s Materials Concurrency approach can do for Additive Manufacturing what it did for the Apple Watch. With our involvement and an investment in 2012, Apple applied QuesTek technology to develop critical materials for the Apple Watch. The watch hit the market in April 2015; throughout that year, 15 million units were sold, and 50+ million units were sold each year between 2016 and 2019, generating an incremental $1.5 billion in revenue each year. The ‘right’ alloy was critical to launching the watch and, at the end, investment in QuesTek’s Materials Concurrency led to a speedy developmental cycle and billions of dollars in fast-growing revenues.
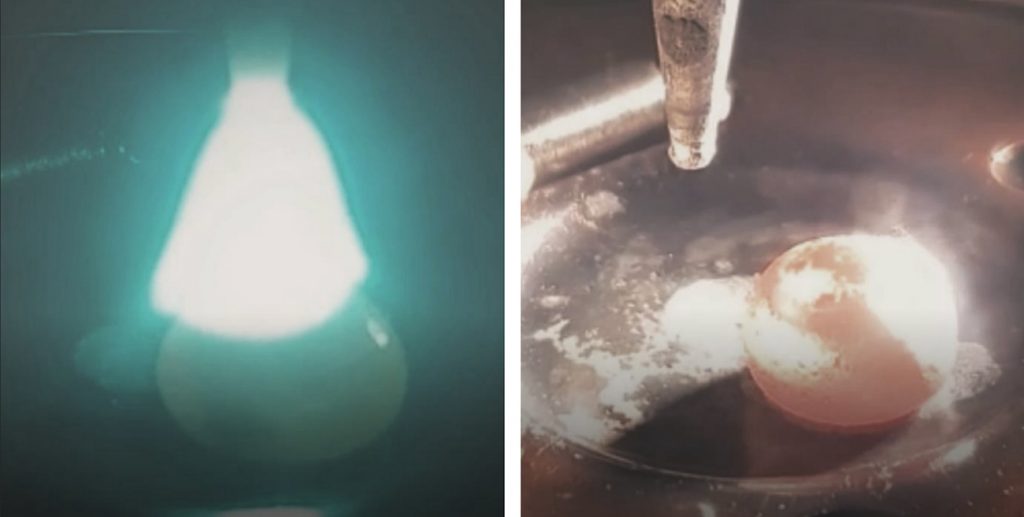
Accelerated insertion of materials
The incorporation of new materials into engineered products – particularly in mission-critical industries such as aerospace, medical device, automotive or energy – can entail numerous steps and production runs to qualify the material and develop statistically driven properties used by design engineers. Failure to achieve material properties accurately and repeatedly at full-scale production levels can be costly and time-consuming. QuesTek uses Materials by Design® technology and our expert skills in Accelerated Insertion of Materials (AIM) to cut down on these risks and costs.
QuesTek was a key contributor to the AIM programme, a strategic initiative led by DARPA and the Naval Research Laboratory (ONR). The AIM programme produced a new way to develop materials that makes it easier to use new materials in the same amount of time as part of the development and design cycles. The AIM programme has achieved the following results:
- Made it possible to set material requirements that are design driven by tightly linking design and material activities and tools
- Provided designers with early material properties, with confidence bounds, throughout the development cycle
- Allowed users to control material performance, producibility, and cost
- Lowered the risk of using a new material while also reducing the cost and time needed to produce data
An exemplary client success story of Accelerated Insertion of Materials is the US Navy carrier aircraft landing gear hook shanks. Under a contract with the Navy, QuesTek designed, produced, qualified, and placed the certified products, made of QuesTek’s proprietary Ferrium® M54 alloy, into flight operations on the Navy’s T-45 trainer aircraft, in a third of the time of a typical design-to-flight cycle (in approx. seven years vs twenty years). The hardness and toughness of the new gear enabled more than two times more landings on the platform, saving millions in maintenance costs and preventing numerous accidents, loss of aircraft (a T-45 costs $17.2M each), and injury or loss of young aviators.
Proprietary materials
QuesTek has thirteen US Patents for ICMD advanced alloys and five US Patents for ICMD advanced alloys used in Additive Manufacturing. It also currently has fourteen US patents pending for additional novel materials. We mostly license our proprietary materials to leading material manufacturers, but we have also shown that we can build and manage custom supply chains for specific, high-performance applications of our proprietary materials for both corporate and government end-use clients. QuesTek’s AM-specific alloys include:
- An AM aluminium alloy that maintains high strength at temperatures over 250ºC for extended life (e.g., 1000 hours at temperature)
- An AM aluminium alloy that has room temperature strength on the order of 7000 series aluminium alloys (without ceramic particle dispersion which can reduce fatigue strength)
- A true ‘build and go’ 17-4 PH AM stainless steel alloy, which under proper build parameters, meets wrought 17-4 PH properties without requiring post-processing solution treatment or age
- A higher-strength Nickel 718 alloy
- Nickel aluminium bronze
- A carburisable Ferrium C64 for gears and other high-toughness, high-wear applications
- HEAs (high entropy alloys)
- Gradient Niobium AM alloy compositions
- Equiaxed, isotropic titanium alloys
Conclusion
Thanks to remarkable breakthroughs and major advances over the previous decade, QuesTek’s Integrated Computational Materials Design provides a unique designing and manufacturing paradigm for accelerating the research and development of innovative materials. When combined with advances and improvements in Additive Manufacturing, ICMD has been proven to create a design and manufacturing paradigm shift in which digital twins make it possible to design materials faster, cheaper, and better suited to their application.
Contact
Keith Fritz, Director of
Solutions Architecture
[email protected]
Severine Valdant, Chief Sales &
Marketing Officer
[email protected]
QuesTek
1820 Ridge Avenue, Evanston,
IL 60201, USA