Binder Jetting and FDM: A comparison with Laser Powder Bed Fusion and Metal Injection Moulding
In recent years there has been a surge in interest in the use of Binder Jetting and Fused Deposition Modelling for the production of low to medium volumes of metal additively manufactured parts. In the following report, Maximilian Munsch, Matthias Schmidt-Lehr and Eric Wycisk of Ampower GmbH & Co KG, Hamburg, Germany, review how the success of Metal Injection Moulding has enabled these technological innovations. They also compare some of key properties and costs to enable designers to better understand the readiness of these technologies for commercial production. [First published in Metal AM Vol. 4 No. 3, Autumn 2018 | 20 minute read | View on Issuu | Download PDF]
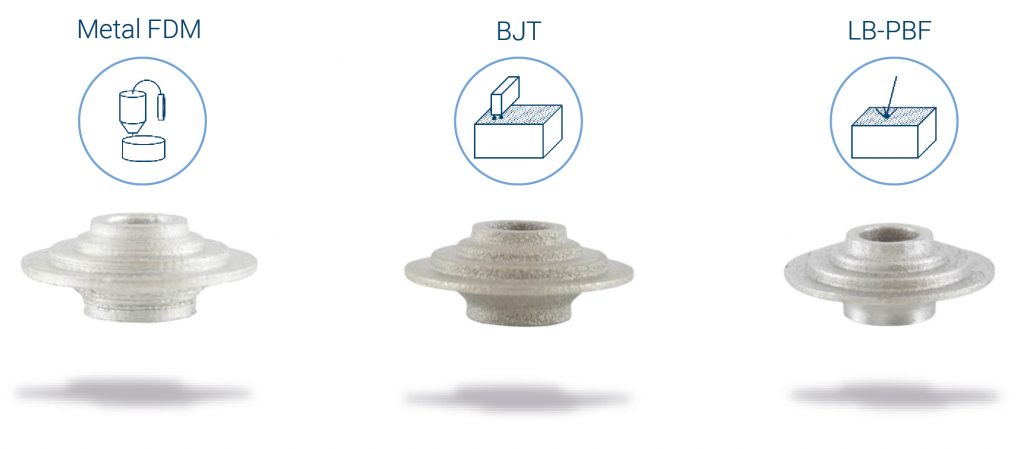
As a technology, Additive Manufacturing can look back on a history of over thirty years. Beginning primarily as a prototyping technology, it has over the last five years gained tremendous momentum for the production of functional parts in a wide range of industrial applications. For many highly demanding applications, Laser Powder Bed Fusion (LB-PBF) has become the dominant Additive Manufacturing technology. However, with the development of metal AM technologies that rely on a sintering process as a final production step, this may be about to change. The technology of fundamental importance to the development of these new processes is Metal Injection Moulding (MIM), hence frequent references to ‘MIM-like’ AM processes.
Together with a number of industry partners, Ampower is currently evaluating whether these sinter-based metal AM technologies can live up to expectations and become a serious competitor to LB-PBF. The industrial partners for this investigation are Alliance MIM, DB Fahrzeuginstandhaltung GmbH, C. Illies & Co. Handelsgesellschaft mbH, Lufthansa Technik AG, citim GmbH, Porsche AG, SLM Solutions Group AG and Yxlon International GmbH.
The aim of the investigation has been to determine the technology readiness level of Binder Jetting and metal Fusion Deposition Modelling (FDM), as well as identify the potential and limitations of these technologies when compared to Metal Injection Moulding and LB-PBF. Whilst the complete report based on the investigation will give a more detailed look into these new metal AM technologies, this article presents key findings that will help guide prospective users to identify the right technology for a specific application.
A diverse range of metal Additive Manufacturing technologies
As of today, there are at least eleven major metal Additive Manufacturing technologies. Each technology has its specific advantages and limitations regarding part design, mechanical properties and costs. This leads to ever more complex decisions when it comes to the choice of technology for a given application.
The technology map shown in Fig. 2 aims to classify these different metal AM technologies whilst taking the ISO/ASTM 52900 standard into account. Most of the processes can be classified into melting or binder-based sintering technologies. While sintering always comes with a binder component and a two-step manufacturing process, melting technologies use powder or wire in a single-step process to directly produce the part.
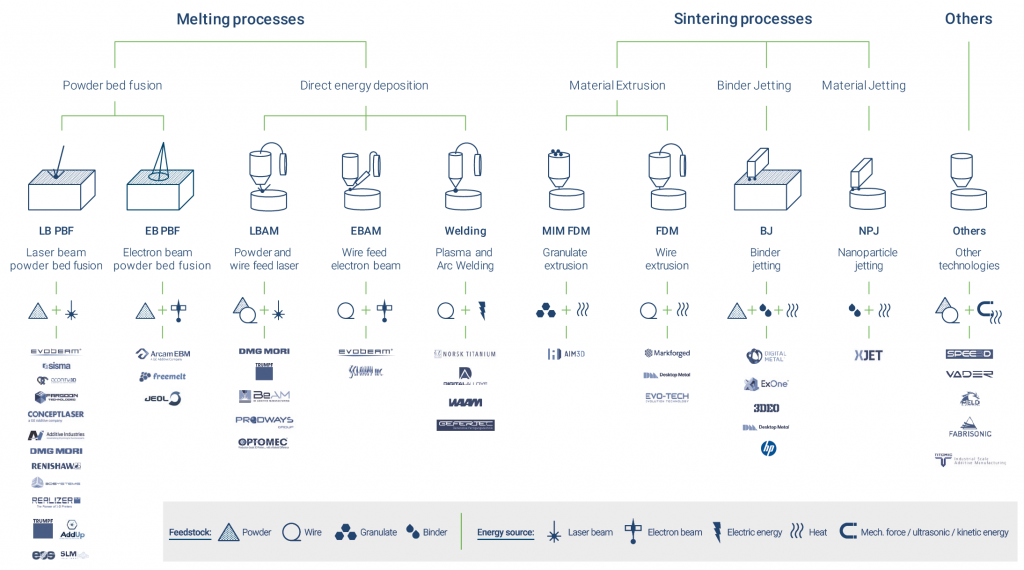
Classification of the different melting/fusion technologies can be accomplished by distinguishing the material deposition method (direct or powder bed) and the energy source:
- Laser beam
- Electron beam
- Electric energy
Sinter-based AM processes require a debinding and sintering step and therefore all have heat in common as the main energy source. They are also classified by the material deposition method and further distinguished by the type of feedstock:
- Conventional MIM feedstock (granules or rods)
- Wire made of polymer binder and metal powder
- Metal powder plus fluid binder
- Binder dispersion with nano metal particles
The evolution of an eighty-year-old technology
Conventional Powder Injection Moulding (PIM) technologies, incorporating the use of a metal or ceramic powder, mixed with a polymer binder and then a debinding and sintering step, are firmly established in today’s manufacturing environments and have a far longer history in industrial applications than Additive Manufacturing. Dating back to the late 1930s and early 1940s, the precursor to what we now know as Ceramic Injection Moulding (CIM) used ceramic powder and a binder to manufacture products such as spark plug bodies. In the 1970s the technology was significantly enhanced with new, more capable binders, and the use of metal powders was investigated. This work led to the birth of Metal Injection Moulding, for which the first patent was filed in 1976. The early cost of MIM parts, however, was extremely high and the process more complex in comparison to competing casting technologies. The subsequent development of more sophisticated binder materials and debinding processes brought improved process stability and easier access to the technology, leading to strong global growth. Today, total global sales of metal injection moulded parts are valued at over €2 billion, with a compound annual growth rate of between 10 and 20%. MIM applications are dominated by complex components for consumer electronics, medical devices, orthodontic applications, firearms, automotive components, watches, jewellery and power tools. Part sizes typically vary between 5–50 mm, however some are in the micro size range and weigh just 0.02 g, whilst others can weigh more than 500 g. The MIM process is based on three steps (Fig. 3):
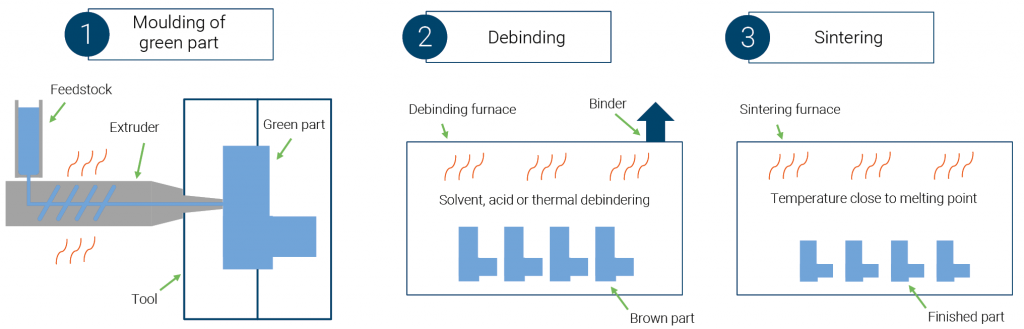
- The forming of a green part by injection moulding with a premixed feedstock (metal powder compounded with a polymer binder)
- The removal of the main binder by a thermal, acid or solvent process to create brown part
- The removal of the ‘backbone’ binder and the sintering of the ‘brown’ part at high temperature
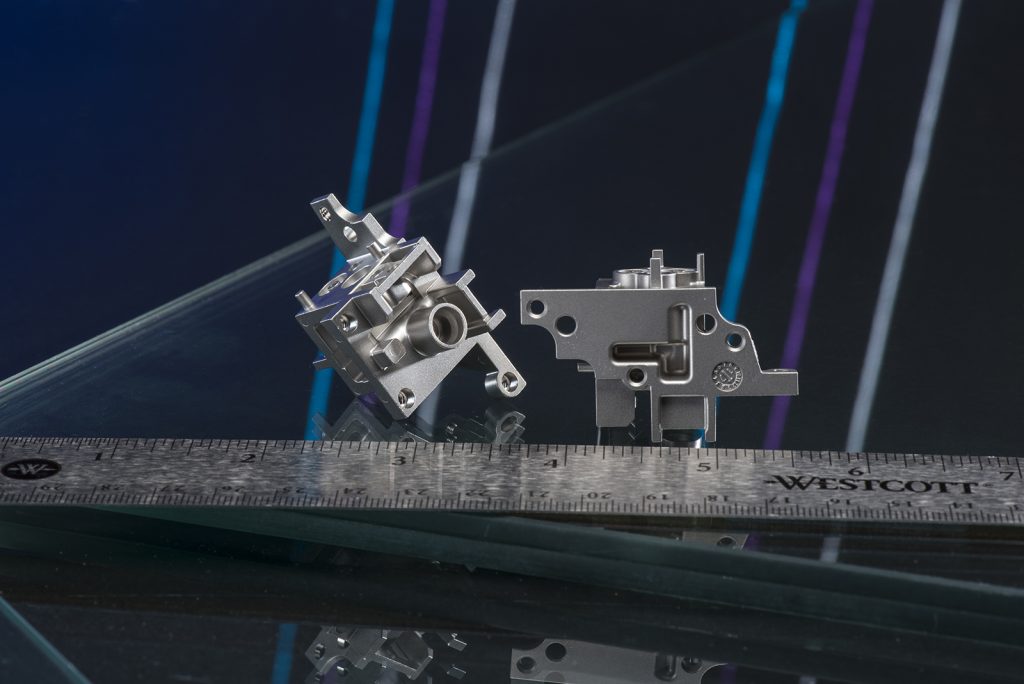
Two recent award winning MIM parts that demonstrate the technology’s capabilities for net-shape high-volume production are shown in Figs. 4-5. Based on the recent success of sintering technologies in general, and MIM in particular, several companies have developed sinter-based metal AM technologies. These new AM technologies share steps 2 and 3 with Metal Injection Moulding. However, the forming of the green part uses the layer-on-layer manufacturing principle of Additive Manufacturing instead of injection into a mould. Nevertheless, the actual material deposition method used to create the green part can vary greatly from company to company.
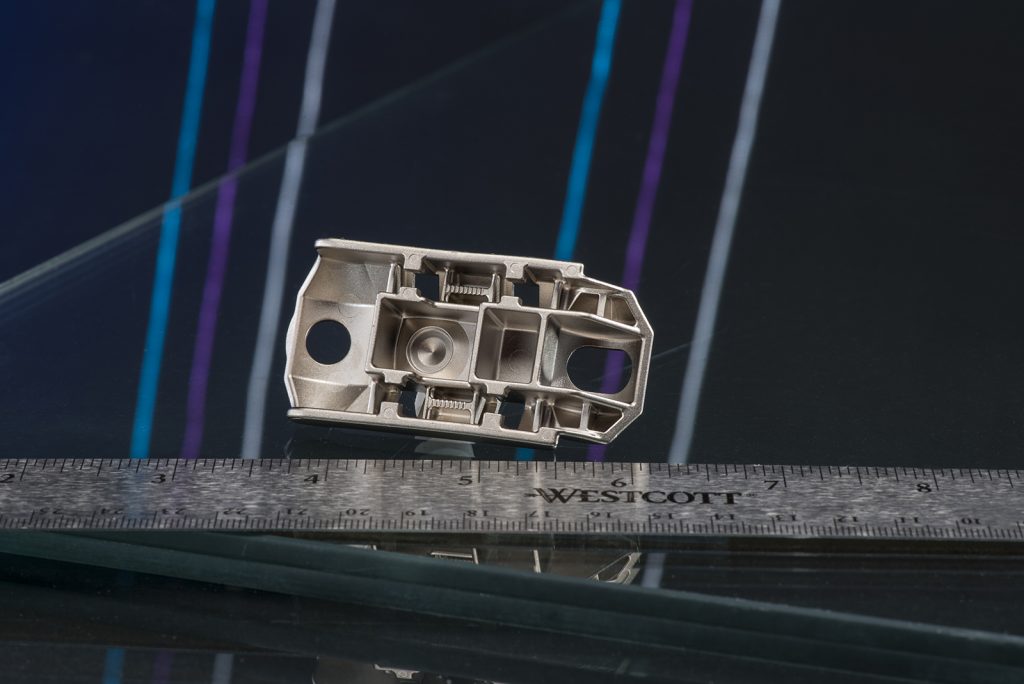
Technology principles
Sinter-based metal AM technologies can be classified into metal FDM and Binder Jetting processes. Both are currently available from several system manufacturers. While Binder Jetting has its roots in the Additive Manufacturing of sand cores, metal FDM is based on conventional FDM technology.
Metal FDM
Metal FDM uses wire, MIM feedstock or rods as the base material (Fig. 6). This raw material feedstock is a compound of metal powder and polymer binder. While the machine principle is very similar to polymer FDM systems, the key difference lies in the extrusion nozzle, which has specific characteristics for applying the metal-loaded feedstock.
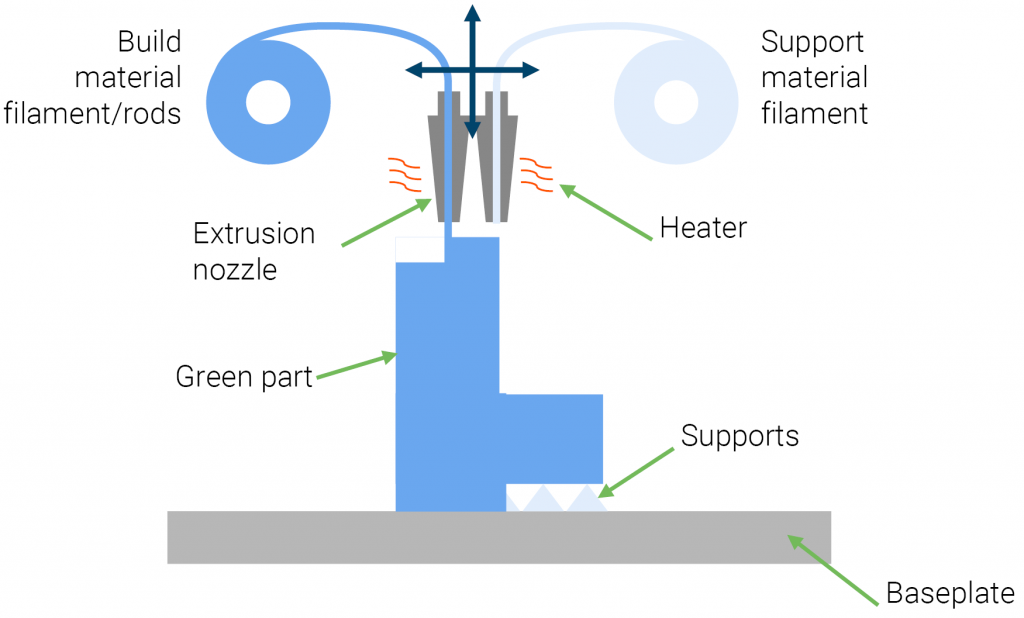
With AIM3D, Desktop Metal, EVO-Tech and Markforged, four major suppliers are currently offering metal FDM printing systems. Their business models vary as they each cover different aspects of the vertical value chain. While Markforged offers proprietary material solutions and debinding/sintering solutions alongside their AM system, AIM3D and EVO-Tech use metal filament and MIM feedstock that is readily available on the market from established suppliers, such as BASF. Furthermore, they use common debinding and sintering process equipment offered by a number of tried and tested MIM furnace manufacturers.
Binder Jetting technology
Binder Jetting is based on an MIT-developed technology which originally focused on sand casting forms. After its initial development, ExOne developed the first metal process based on Binder Jetting, with Höganäs’s Digital Metal following with its own system. Desktop Metal is currently publicising its binder jet-based Production System and, in September 2018, HP unveiled its first binder jet metal AM system.
The technology principle is still based on the original MIT patents, which refer to a powder bed of sand or metal powder on which binder is locally applied. This fluid binder solidifies by applying heat (Fig. 7). A slightly different approach is described by 3DEO. Here, the binder is applied on the complete powder bed area followed by a milling process of the hardened layer with multiple machining spindles to create the contour of the part in each layer.
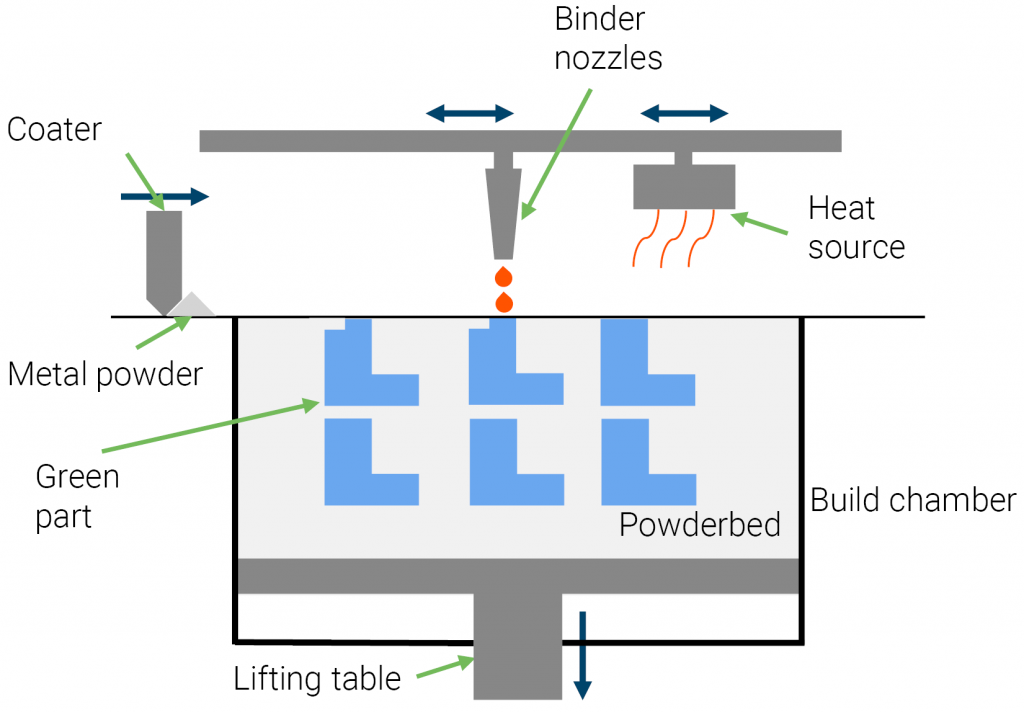
Engineering focus: material, quality, design
Material availability and performance is a key enabling factor for new Additive Manufacturing applications. At the moment only LB-PBF offers a wide range of materials, with alloys based on aluminium, titanium, nickel, steel and precious metals, as well as other materials in development such as magnesium. In theory, any weldable material can be processed by LB-PBF. A multitude of publications on the mechanical properties of all these alloys means that engineers can be confident in their expected characteristics and ultimately results in a higher acceptance of LB-PBF over less-researched technologies.
As of today, 17-4PH and 316L stainless steel are readily available for Binder Jetting and metal FDM technologies. Digital Metal also offers parts in titanium alloy Ti-6Al-4V. In principle, all known MIM alloys can be used for sinter-based AM technologies. Copper and carbide materials pose particularly interesting opportunities for future applications, since they have certain limitations in LB-PBF. On the other hand, aluminium alloys will remain challenging for the new Additive Manufacturing processes due to the difficulty of sintering aluminium.
Material properties
For this study, stainless steel alloys 316L and 17-4PH were chosen to determine the material characteristics. These alloys are commonly available for the LB-PBF, MIM, metal FDM and Binder Jetting processes and therefore offer the best property comparisons. The obtained results are based on over fifty specimens from eight different system suppliers. The complete test program includes tensile testing, hardness and surface roughness measurements as well as micrograph and µCT analysis.
This report will focus on the hardness measurements and density analysis, which represents a major quality factor for metal parts. A high material density is paramount for good material characteristics such as tensile strength and fatigue behaviour. Also, with design restrictions in mind, a certain density is required for all components which carry fluids or gases. In these applications, low density leads to the need to increase wall thicknesses to achieve the necessary impermeability of the part.
For density analysis, six sections of each technology were analysed by light microscopy. The analysis includes micrographs from several Binder Jetting and metal FDM system manufacturers as well as MIM and LB-PBF specimens. The results show significant differences in pore size, shape and distribution between the technologies (Fig. 8). For all sinter-based technologies, the most likely cause of this is the different debinding and sintering strategies and not the specific Additive Manufacturing mechanism. It is worth mentioning that the density of all analysed MIM samples exceeds the standard MIM as-sintered density range of 95–97%. This however is unsurprising, as the selected supplier targets demanding applications with high performance requirements.
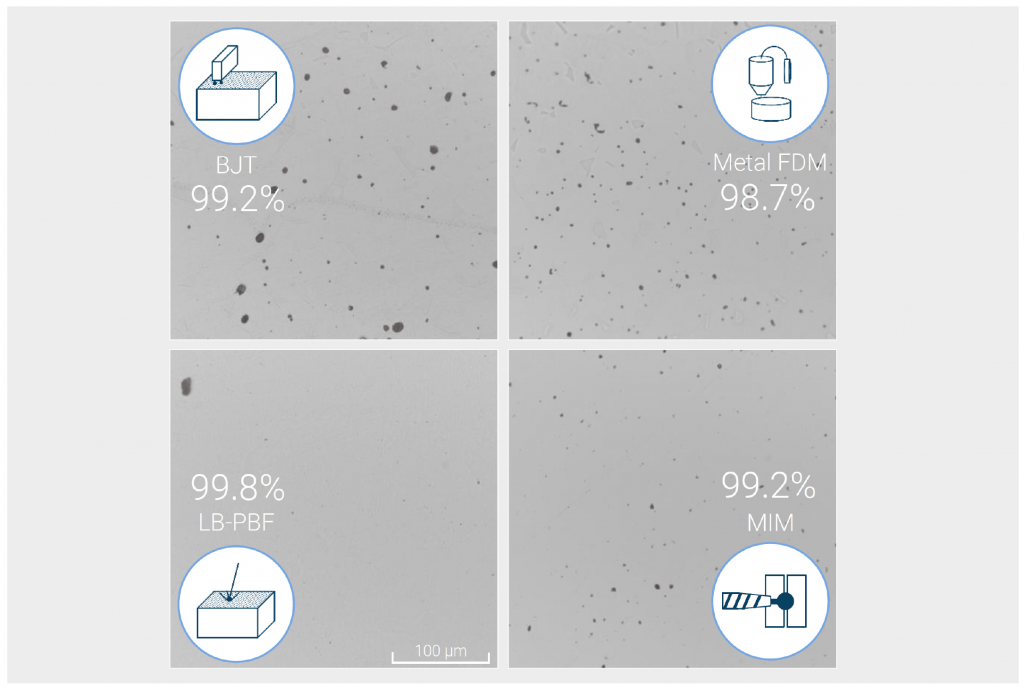
To minimise the influence of sintering temperature and other process-related factors on the mechanical properties, and to increase the comparability of processes, all specimens were additionally heat treated after sintering in identical cycles. All specimens were solution treated at 1040°C for 1 h in a vacuum. The 17-4PH specimens were additionally hardened to H1025 at 550°C for 4 h in atmosphere. Figs. 9-10 show the resulting hardness measurements. It is evident from these results that sinter-based AM technologies achieve values close to the requirements for metal injection moulded materials as defined in ISO 22068 for 316L, and exceed the standard value for H1025 hardened 17-4PH.
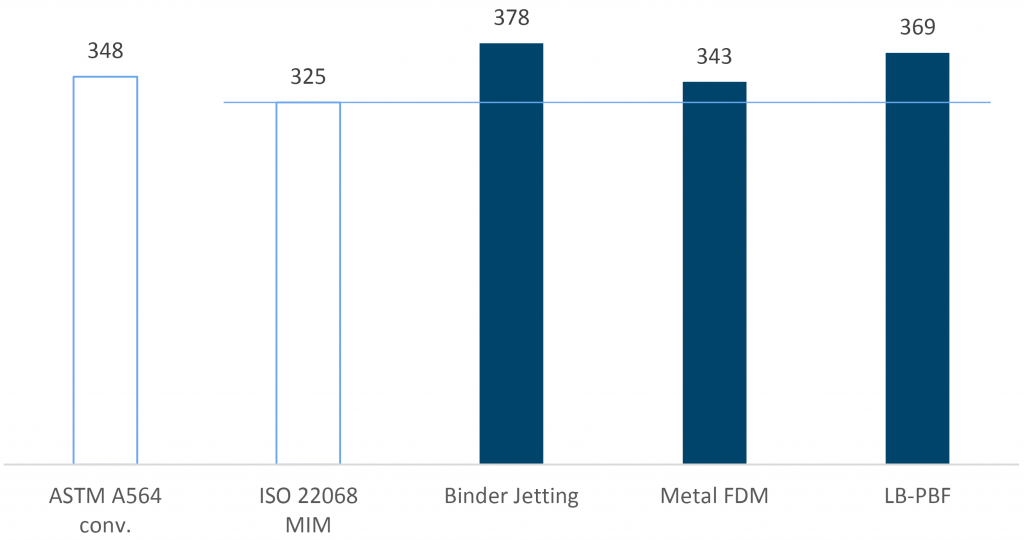
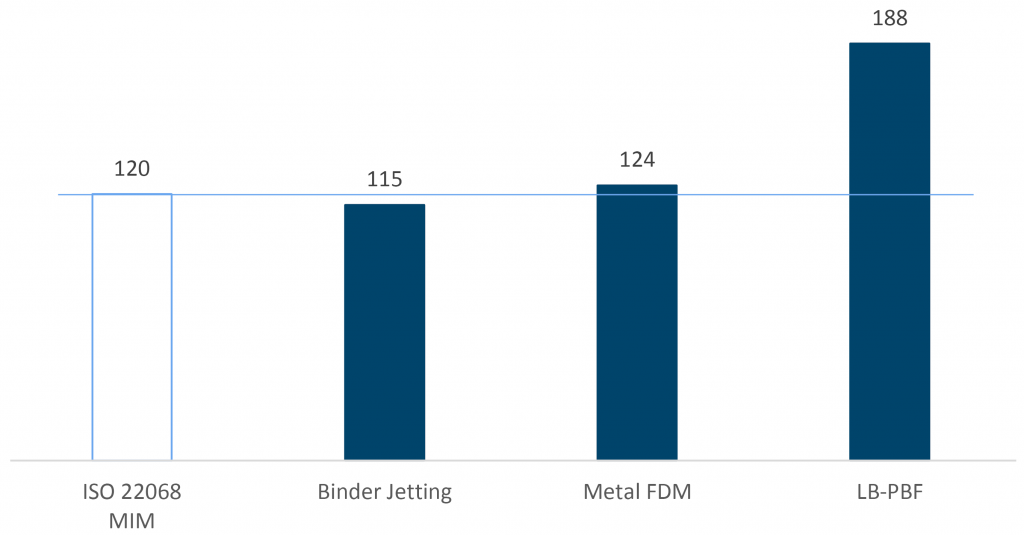
Design
Additive Manufacturing technologies offer superior design possibilities compared to conventional manufacturing processes; however, residual stresses and the need for support structures add restrictions to the freedom of design when using AM technologies such as LB-PBF.
While Binder Jetting requires no supports during the build process, the sintering step may require additional supports to prevent deformation of the part. An alternative can be achieved by using ceramic ‘setters’ that hold the component in place and support areas that are prone to deform during sintering. This would require higher production volumes to amortise the associated costs.
Regarding part size, MIM parts are characterised by rather small component dimensions of typically 50 mm or smaller. The reason for this limitation lies in the debinding and sintering process. From a practical point of view, debinding limits the maximum material thickness to 5–10 mm. Exceeding this value increases the debinding time exponentially or makes complete debinding impossible. Additionally, sintering requires temperatures close to the material melting point, which can cause deformation when large masses or geometric complexity exist. During the sintering phase, the part undergoes the most significant shrinkage and a suitable surface is therefore required to allow this movement.
All these MIM restrictions also apply to sinter-based metal AM technologies. With dimensional distortion during sintering difficult to predict, one of the major advantages of Additive Manufacturing – the ability to deliver very low volumes or one-off parts – is negated. Developing a sinter-based process for complex parts requires several test loops to control any distortion during sintering. This limits these AM technologies to either simple part geometries or trial and error loops for complex parts.
Surface
Another important factor in metal Additive Manufacturing is the surface quality, as high accuracy and low surface roughness might make additional mechanical post processing unnecessary. In this regard the surface finish of parts made by LB-PBF is often compared to the surface quality of cast parts. While functional surfaces must be post-processed, free form surfaces can be kept as-built or simply sand blasted. In MIM, as-sintered quality usually fulfils the requirements for functional surfaces. The surface roughness, as well as the repeatability of dimensional accuracy, allows the production of highly precise parts without mechanical post-processing. If all the functional surfaces cannot be achieved in the moulding process, it is common to process the parts in the green state. Milling operations are significantly easier while the part is unsintered and the same holds for sinter-based AM parts.
This study analysed the arithmetic average surface roughness in the as-sintered state by tactile measurement. The values were obtained by measuring the specimens’ surfaces in the zx- and xy-plane. The mean value of both measurements allows a technology comparison. However, especially for metal FDM, the surface roughness depends significantly on the build direction (Fig. 11).
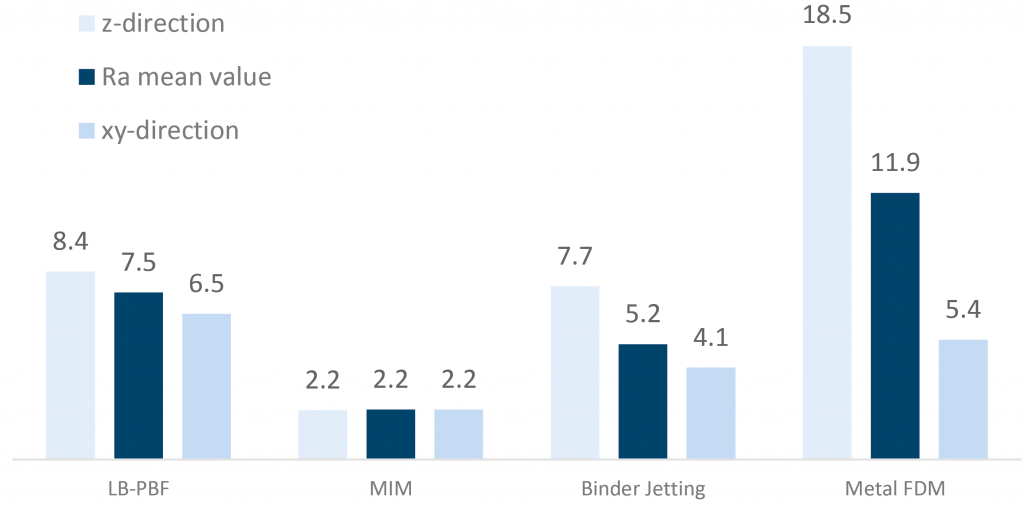
Business focus: cost and value chain
In the case of LB-PBF, metal Additive Manufacturing is characterised by high machine cost, low production speed and high material cost. Thus, not every part that is technically feasible is financially viable. Sinter-based metal AM technologies promise to change this and lower the cost for metal AM parts significantly.
Material cost
The cost for metal powder suitable for LB-PBF ranges from €40 to €400 per kg, depending on the alloy. For stainless steel, the cost is at the lower end, between €40 and €80 per kg. As with LB-PBF, current Binder Jetting technologies use spherical powder particles; however, many of the new technologies, such as that of Desktop Metal, use conventional MIM powders. Since the cost for stainless steel MIM powder such as 17-4PH is only €5–10 per kg, this offers great potential for reducing material cost by 80–90%, although the cost of the binder material must also be added.
For metal FDM the feedstock consists of wire, granulate or rods made of metal powder and polymer binder compound. Here, the cost of binder is already included. However, the spread in feedstock cost is significant. While granulates are based on conventional MIM feedstocks and range between €15–18 per kg for 316L, filaments such as BASF’s Ultrafuse 316LX are priced at around €200 per kg. However, similar metal filaments are also sold from other suppliers for €100 per kg. While Binder Jetting and granulate based metal FDM are leading to massive cost reduction on material, other metal FDM technologies result in even higher feedstock pricing than LB-PBF.
System investment
The typical investment for a twin laser LB-PBF system is around €500,000 and the depreciation and consumables add up to an average hourly machine rate of €30-40. Single laser systems are at the lower end of this range while the newest quad laser systems cost up to €55 per hour.
Binder Jetting systems which offer comparable build envelopes in the range of edge lengths of 300 mm have similar investment cost covering only the part build process, excluding the debinding and sintering systems. Additionally, costs for consumables and maintenance have little impact in both technologies which results in similar machine hourly rates for Binder Jetting. Future Binder Jetting machines are expected to carry an even higher investment cost of around €1 million, excluding debinding and sintering, but promise a further increase in productivity.
For metal FDM systems, the cost for investment, maintenance and consumables is considerably lower. Focusing only on the build process, machine cost ranges between €60,000–100,000 resulting in hourly rates of €5–8.
Production cost
The production cost for the series production of the AM demonstration part (Fig. 1) has been calculated by the production speed in cm³/h and machine hourly rate for the respective AM system (Fig.12). Additional costs from any necessary mechanical post-processes as well as inspection efforts for quality assurance are excluded.
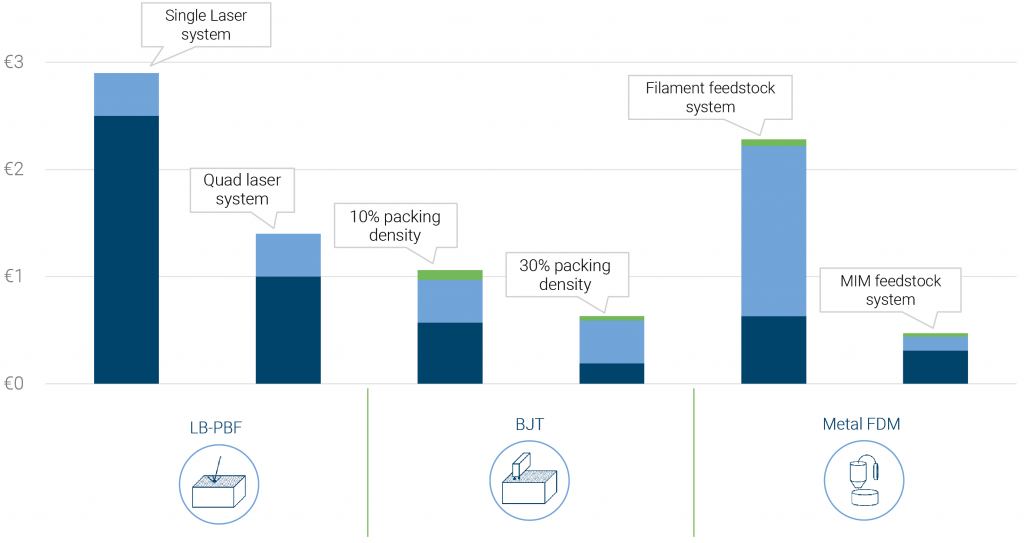
Comparing the production speed proves to be challenging. While LB-PBF and FDM systems can be quantified by their build rate (volume over time in cm³/h), the build rate of Binder Jetting processes largely depends on the packing density. Build jobs of the same height usually take the same amount of total build time independently from the aggregated part volume.
Analysis shows that LB-PBF production speed mainly depends on the number of lasers working in parallel in the build chamber. Based on the mentioned hourly rate, the resulting cost per volume varies between €1-3 per cm³ for stainless steel. For metal FDM, the layer thickness is the key factor to increase the build volume per time. By increasing this parameter, the production rate increases but also leads to a significant reduction in resolution and surface quality. As previously mentioned, the cost of feedstock also varies depending on the system. Taking these parameters into account, the resulting cost for stainless steel is estimated to be between €0.5 and €2.5 per cm³.
Production speed in Binder Jetting is calculated from the total build job time and packing density. The packing density reflects the sum of all part volumes referred to the complete build envelope. Packing density is typically 10% for mixed build jobs with different parts and 30% for optimised stackable parts in a large volume production. Build times are between twenty and forty hours. Considering the claimed improvements in the technology in the next few years, the build envelope will increase while the total build time reduces to ten hours thanks to single pass jetting. With the systems available today, the cost of a build with stainless steel is between €0.5 and €1 per cm³.
Sintering process
Binder Jetting and metal FDM printing processes are always followed by an additional debinding and sintering process. The costs for this largely depends on the specific debinding and sintering technology used by the system supplier. In MIM, catalytic debinding and sintering processes dominate, thanks to their high productivity and good process control. On the other hand, the catalytic process requires a high level of equipment investment, process knowledge and the use of hazardous consumables such as nitric acid.
To reduce complexity, some sinter-based metal AM technology vendors use a one-step thermal debinding and sintering process. For components with wall thicknesses of up to 3-4 mm, this takes about sixteen hours in a typical furnace with a volume of 50,000 cm³. The hourly rate for a thermal debinding and sintering furnace ranges between €13 and €23, with the lower estimate being for a typical debinding operation and the higher figure representative of a typical sintering operation. This figure is based on a calculation that includes utilities and consumables such as electricity, process gas, the cost of a standard furnace with deprecation over five years with an annual run time of 6,000 hours, and furnace maintenance. Excluded from the calculations are labour, infrastructure and overheads, etc. Assuming a packing density of 10%, the cost for thermal debinding and sintering is estimated at €0.08 per cm³. In contrast, the cost for catalytic debinding and sintering adds up to about €0.28 per cm³.
The impact of sinter-based Additive Manufacturing technologies on end users and supply chain
The end user
Component manufacturers now have more options than ever when it comes to choosing the right technology for their application. However, this choice also challenges engineers to develop knowledge of several different production technologies. In the past few years, casting and forging engineers have had to adopt and develop knowledge about LB-PBF. Now, the technologies of Binder Jetting and metal FDM offer an even wider choice. More than ever, the knowledge about possibilities, limitations and characteristics of the different manufacturing technologies becomes a crucial factor for engineers of tomorrow. Fig. 13 shows a technology map to support the choice of technology based on manufacturing volume and part complexity.
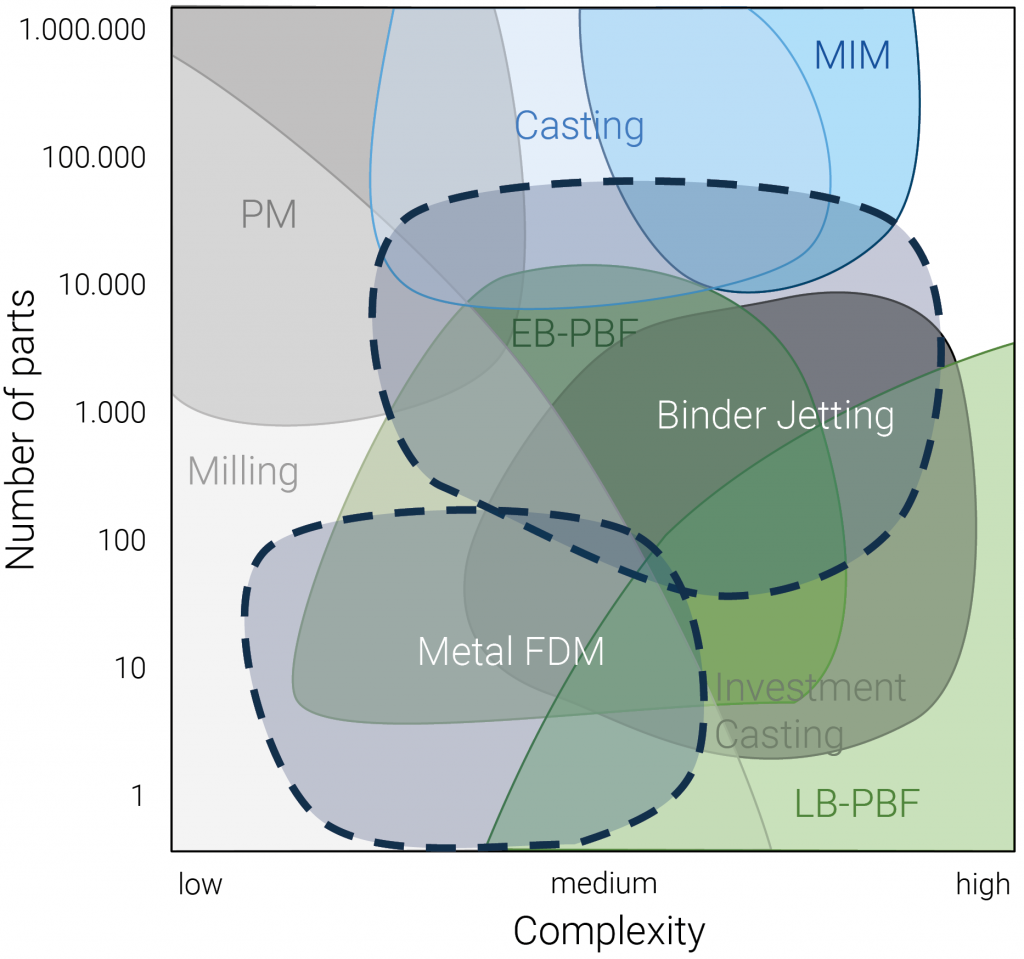
At Ampower we expect the main impact for Binder Jetting to be seen in the higher production volume applications as, for example, seen in the automotive industry. Metal FDM technology will enable applications in the machine industry, especially when it comes to low production volumes, prototypes or jigs and tools. Due to the high requirements on material properties, we expect it to be unlikely that aviation and implant manufacturers will adopt sinter-based AM technologies for highly loaded parts.
The supply chain
The AM supply chain is bound to experience changes with the adoption of these emerging technologies. Starting with material feedstock, today’s metal powder suppliers face new markets in Binder Jetting and metal FDM technologies. However, both technologies will have different requirements for their products.
We expect existing electron and laser beam PBF system manufacturers to further focus on demanding, high-end markets such as medical and aviation industry. The superior material properties and a high technology readiness level of PBF systems will also keep binder technologies at a distance in these high-end sectors. Mass markets such as automotive, however, will gradually shift their focus from LB-PBF to Binder Jetting. The prototyping and tooling market will quickly adapt to the cost potentials of metal FDM.
The major limit of binder technologies will remain in the part size and complexity limitations of the sintering process. Here, manufacturers of sintering equipment and toll sintering operations will experience a significant growth in interest in their products and services. Furthermore, we expect that many conventional MIM producers will integrate sinter-based AM technologies into their portfolio.
Authors
Maximilian Munsch, Matthias Schmidt-Lehr and Eric Wycisk
Ampower GmbH & Co. KG
ZAL TechCenter
Hein-Sass-Weg 22
21129 Hamburg
Germany
Tel: +49 40 99999 578
Email: [email protected]
www.am-power.de
This report is based on a forthcoming publicly available study that offers a detailed look into these new metal AM technologies. The full study will be available through www.am-power.de.
Ampower is a leading consultancy in the field of industrial
Additive Manufacturing. The company advises its clients on strategic decisions
by developing and analysing market scenarios as well as compiling technology
studies. On operational level, Ampower supports the introduction of Additive
Manufacturing through targeted training program as well as identification and development
of components suitable for production. Further services include the setup of
quality management and support in qualification of internal and external
machine capacity. The company is based in Hamburg, Germany.