Alloys by Design: The future of materials for Additive Manufacturing
There is no shortage of Additive Manufacturing machines humming away in research laboratories, producing test pieces and exhibits for trade shows, but the hard truth is that relatively few are making components for serial production. In part, this is because the world is still waiting for materials which enable the technology to fulfil its true potential. In this article, Rebecca Gingell and colleagues from OxMet Technologies, Oxford, UK, explain how the company is approaching the design of novel alloys for AM, and reflect on its progress so far. [First published in Metal AM Vol. 5 No. 4, Winter 2019 | 15 minute read | View on Issuu | Download PDF]
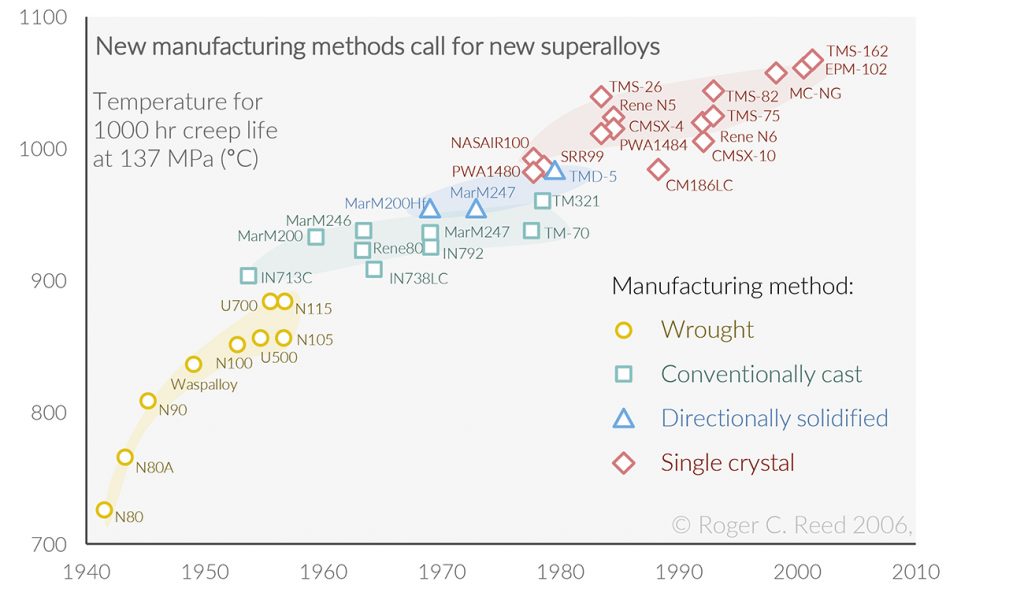
For five thousand years, whenever a new manufacturing method was invented, new alloys were never far behind. The techniques used to develop these alloys have evolved from the enthusiastic addition of arsenic to copper to make bronze, to the use of super-precise probes to assess atom distribution in modern alloys. Ultimately, however, the performance of components has been highest when they’re made from alloys which have been tailored to the specific demands of the manufacturing process by which they are made. Additive Manufacturing is not likely to be any different.
While developers are having some success using legacy alloys in Powder Bed Fusion (PBF) and wire-based AM, the performance of AM components can be lower than cast or forged parts when produced from the same alloys, and many high-performing alloys are not amenable to the repeated melting entailed by most AM processes. A new generation of alloys is required; not just to overcome these challenges, but also to take advantage of the new alloy design opportunities afforded by AM technology.
OxMet, based in Oxfordshire, UK, is the developer and operator of a proprietary computational platform for the design and optimisation of new alloys. The company is currently commercialising a new series of nickel alloys, the ABD®-XAM series, which has been designed from a clean sheet for the specific demands of AM. The range promises a combination of performance and AM processability unlikely to be matched simply by empirical modification of existing alloys. This article provides an overview of the ABD platform and how it can be applied to the development of alloys for AM, and reports on some of the results achieved to date.
New alloys: A numbers game
Advanced manufacturers of alloys are generally able to control the proportions of elements in alloy compositions to an accuracy of 0.1%. Even small changes in the concentrations of different elements can lead non-linearly to large changes in behaviour and performance. Hence, the number of distinct alloys which could be made from a 10-element nickel superalloy, for example, could be as high as 1020. Even for a comparatively simple alloy system, such as a ternary titanium alloy, there could be as many as 250,000 compositions exhibiting significant differences.
The size of these numbers, and the complex interaction between composition and process which determines alloy performance, has historically made alloy design difficult to approach analytically and computationally. The improvements of the past seventy years have therefore largely come about through increasingly well-informed empiricism: designers take a familiar alloy, modify the composition using insights from research and their own experience, and test samples to see if their efforts have been successful.
The relatively high cost of this approach has been a factor for conservatism, particularly in industries producing large or safety-critical parts – shortcomings which don’t manifest themselves until a late stage of qualification in a large component can cost millions in waste.
In recent years, however, two key developments have occurred: physical models for predicting composition-dependent properties have improved, and computing horsepower has increased significantly. These changes have rendered the alloy design process more and more tractable by analytical approaches. The Oxford University research group led by Professor Roger Reed, from which OxMet Technologies emerged, has been one of the leaders in the growing field of computational alloy design.
OxMet’s Alloys by Design platform
Fig. 2 Structure of OxMet’s ABD platform (Courtesy OxMet) [click image to enlarge]
OxMet’s ABD software platform is an optimisation tool for identifying new alloy compositions. It uses physical – and in some cases physical-empirical – models to analyse and rank millions of different alloys simultaneously on a range of economic and performance metrics. This enables the optimal alloy for the specific needs of a given application to be rapidly identified (Figs. 2-3).
Fig. 3 OxMet’s ABD software platform (Courtesy OxMet) [click image to enlarge]
The alloy design process begins with a consideration of the trade-offs in properties associated with the demands of a particular component, and a statement of which performance metrics are ‘target metrics’ – normally strength, creep resistance, or other metrics where one can never have too much performance. Then there are metrics which are ‘threshold metrics’: threshold metrics often include a ceiling elemental cost, and another common example is the lower limit for elongation common to alloy design projects for the automotive industry.
Secondly, on the basis of experience, Oxmet defines a wide alloy space – the palette of allowable major and minor elements for the target alloy, and upper and lower bounds for each element, with zero being the lower limit for elements for which inclusion is optional. OxMet then uses the ABD software platform to model the performance of all possible alloys within that space on the metrics deemed relevant in the scoping exercise, at user-determined intervals for the proportion of each element in the composition space (for reasons of computational economy, OxMet often scans a large space at a relatively coarse resolution, such as 0.25%, before zooming in on an attractive space for finer-grained evaluation at intervals of 0.1%).
The accuracy of the platform is evolving and varies between performance metrics, and some iteration is still occasionally required. But overall, it reduces the time taken to arrive at a new composition from many months, or sometimes years, to a few weeks. After the computational stage, OxMet will produce three or four compositions for testing, with the best of these often adopted as the final alloy.
The platform has further salient advantages, for example for highly multi-dimensional optimisation problems in which developers seek improvement on one or two performance criteria, but require that the alloy is adequate on a large number of others. As an example, developers of nickel alloys for turbine blades may seek improved high-load, high-temperature creep resistance, but also set thresholds for corrosion resistance, oxidation and fatigue.
The ABD platform allows ‘global’ optimisation from a clean sheet. Empirical approaches tend to favour local optimisation starting from a known alloy, whereas the ABD platform allows exploration of an almost limitless alloy space. This is particularly important when developing alloys for completely new manufacturing platforms.
Finally, and of great relevance for investors in materials development, the platform is very helpful in filing strong, broad, commercially valuable patents over compositions, because of the power it provides to discover and rationalise the boundaries of the ‘compositional windows’ within which promising alloys are likely to be found.
Using the ABD platform to design alloys specifically for AM
Alloys optimised for traditional manufacturing processes tend to underperform when used in AM. The precise limitations vary metal to metal; in titanium alloys, columnar growth and anisotropy are problems, while in aluminium alloys, solidification behaviour creates cracks, and in nickel alloys, unstable phases limit performance at high temperatures.
To apply the ABD platform, OxMet has to build physical models which predict an alloy’s susceptibility to the relevant problems. These models can be incorporated in an optimisation exercise in which these susceptibilities can be avoided, while designing for strength, creep resistance, or other performance criteria.
Designing high-performance nickel alloys for AM
The particular challenge of high-performance nickel alloys is that the primary mechanism by which they have historically been made resistant to creep – the introduction of high levels of γ’ phase – leads to cracking of various forms (see Fig. 4).
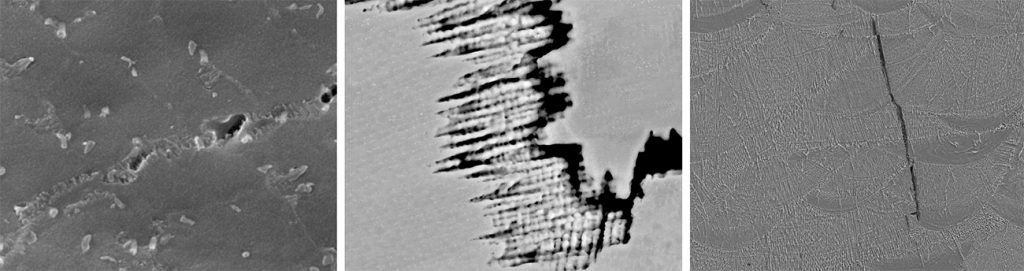
OxMet has built models for susceptibility to each of these mechanisms of crack formation, and has used these models and its model for strength in superalloys to explore an extremely wide alloy space (Fig. 5). This enabled OxMet to identify completely new compositions with a significantly lower propensity to crack during Additive Manufacturing and post-processing than existing γ’-strengthened alloys, but which retain very high levels of strength and creep resistance at elevated temperatures.
Fig. 5 OxMet uses its ABD® platform to predict the processability and strength of millions of alloy compositions, and then filter to identify the optimal trade off (Courtesy OxMet)D software platform (Courtesy OxMet) [click image to enlarge]
Multi-optimisation strength models
A key tactic in achieving optimal results has been using all the levers available to improve mechanical performance, rather than rely only on those which have been used traditionally. For example, while increased fractions of γ’ precipitate have been the traditional means of strengthening nickel alloys, the strength model used by the ABD platform shows there is another lever available: that of increasing the anti-phase boundary energy.
OxMet’s ABD-850AM and ABD-900AM chromia-forming alloys – in as-built form at least – contain higher anti-phase boundary (APB) energies and lower γ’ content than cast alloys designed to operate at similar temperatures. Following the build, the γ’ content is raised through heat treatment if required.
Processability
The charts and images in Fig. 6 indicate the ease with which ABD-900AM can be processed by AM, by comparing crack length and densities between it and various commonly used high-performance nickel alloys. These include IN-718, which is known to be relatively easy to process by AM, and the more difficult-to-process IN939, IN738, and (not shown) M247. ABD-900AM shows similar levels of high-temperature strength and creep resistance as IN738.
Fig. 6 The above charts and images indicate the ease with which ABD-900AM, in comparison to benchmark nickel alloys, can be processed by AM without compromising high temperature mechanical performance (Courtesy OxMet) [click image to enlarge]
Versatility
Interestingly, OxMet has tested processability by PBF with both the 30 µm normally used for the AM of high-performance nickel alloys, and 60 µm layers, and found that, with test pieces at least, fully dense parts can be made from ABD-900AM with 60 µm layers. This could have significance for the cost at which components can be made in serial production.

The ABD-XAM range was developed at Renishaw using a 400AM. However, it has successfully been used on multiple systems with similarly defect-free results, using IN718 process parameters.
Orthopaedic-specific alloys for bone implants
2007 saw the death of Monsieur Mangetout, the Frenchman who consumed a Cessna 150 in 1978. Whilst it is unlikely that this feat prolonged his life, he would certainly have met his iron quota in that year. Unfortunately, Monsieur Mangetout would also have consumed a large amount of vanadium, which happens to be poisonous.
Vanadium is an element in the most common titanium alloy for aerospace, the high-strength, high-stiffness Ti-6Al-4V (Ti64). Despite this toxicity, Ti64 is also the most common alloy for orthopaedic implants – not ideal for a component designed to last for decades. Ti64’s mechanical properties are also sub-optimal. The high stiffness of parts produced in this material, so useful in the Cessna 150, causes stress shielding in bone (Fig. 8), leading to bone resorption and implant loosening. This ‘macro’ stiffness can be countered using mesh structures with high elasticity – but at a micro scale, osteoblasts still find it difficult to grow on the stiff, immobile struts. Finally, Ti64 is anisotropic, yielding varying properties depending on build direction.
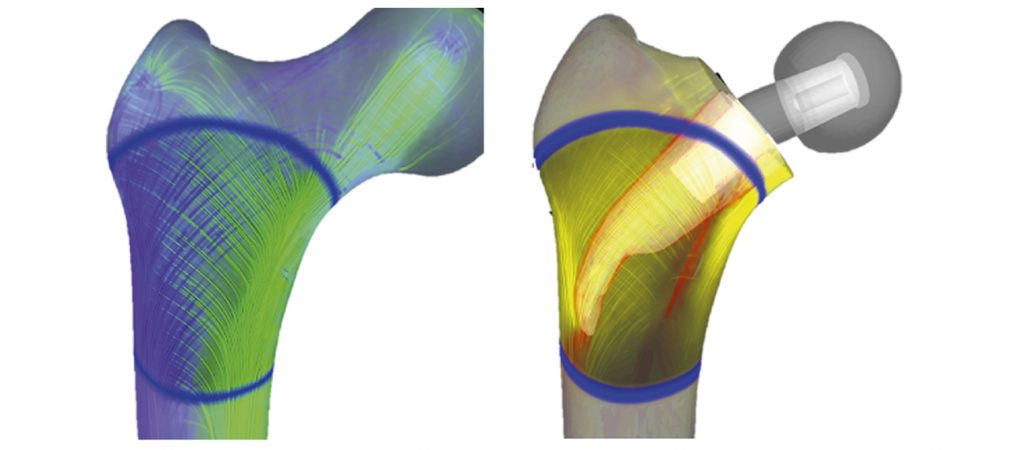
OxMet set out to design a titanium alloy with no cytotoxic elements, with a third of the stiffness of Ti64, and with enough strength to hold up a person. If the alloy could be isotropic with a wide processing window, so much the better. Fig. 9 shows the workflow for the development, testing and manufacturing of a new custom alloy for this type of application.
Fig. 9 Workflow for the development, testing and manufacturing of a new custom alloy for medical implants (Courtesy OxMet) [click image to enlarge]
Introducing and commercialising new alloys
OxMet has two commercial models for its alloy development activities: licensing alloys it has developed itself and providing bespoke alloy development services to customers. It has recently licensed its ABD-XAM series of nickel alloys to Aubert & Duval, and the companies are working together to sample and promote powders under Aubert & Duval’s Pearl®Micro brand.
As a would-be licensor of new alloys, OxMet needs to show a strong appreciation of the pathway new alloys need to take to market. Some parts of the alloy market are highly conservative, and have significant barriers even to trial new alloys.
Fortunately, the Additive Manufacturing industry is at an early stage of development when companies are more open to trying new alloys. The fact that the minimum volumes of alloy required to conduct a meaningful test are so much lower for AM than for many manufacturing processes also helps. Equally, the clear limitations of existing alloys are forcing a degree of adventurousness in alloy selection.
However, AM does bring some challenges of its own. AM production cells vary significantly, so that an alloy that can be built well on one machine may perform poorly on another. OxMet gets around this by designing compositions with wide processing windows. There is also large diversity in customer knowledge of AM and confidence with new materials.
Designers of AM components don’t replicate traditionally-made parts; they take advantage of the design freedom afforded by the process. But many designers still specify the previously-used alloy, even though its properties after AM can be radically different. While no company’s new alloy introduction process is the same, the flowchart in Fig. 10 shows the typical introduction cycle for a new alloy, along with some of the pitfalls along the path at which an alloy can fall off a development schedule.
Fig. 10 The typical introduction pathway for new alloys (Courtesy OxMet) [click image to enlarge]
The future of materials for AM – bespoke alloys or all-purpose solutions?
A much-debated question in the OxMet kitchen is whether the future of Additive Manufacturing will be characterised by a few ‘workhorse’ alloys which will be put to multiple uses, or a plethora of different alloys, each matched to a small number of applications, or even a single application.
Those on the ‘workhorse’ side of the argument are led by Dr David Crudden, a veteran of extended aerospace alloy qualification campaigns and Head of Alloy Development at OxMet. This argument points to the high costs of qualifying new alloys in many of the industries where AM is of greatest interest, and the economies of scale which derive from material recycling, manufacture at scale, and a high level of competition between alloy producers.
Those who argue that the future is built in bespoke alloys, led by Dr André Németh, OxMet’s Programme Director and a member of the founding team, argue that the principal virtues of AM are precise customisation and ever-denser functionality, and that there is no reason why AM alloys should be any different. They also note that raw materials account for a much lower proportion of total product cost than for traditionally manufactured parts, and that the atomisation by which the powder feedstock for most AM processes is created is in any case a relatively small-batch process.
Perhaps the conclusion is that different markets will find different answers; for some, qualification costs will continue to be a conclusive factor preventing alloy palette consolidation for some years to come, until opportunities for digital qualification start to bring down the costs of introducing new alloys. In other markets where barriers to new alloys are lower, such as in electronics, a wide range of alloys is indeed likely to develop, and intelligent master alloy and alloy customisation strategies will still allow high levels of recycled alloy use.
Contact
Rebecca Gingell
+44 1865 954 258
[email protected]
Unit 15, Oxford Industrial Park
OX5 1QU, UK