Nanoscale Additive Manufacturing of metals developed at Caltech
September 22, 2023
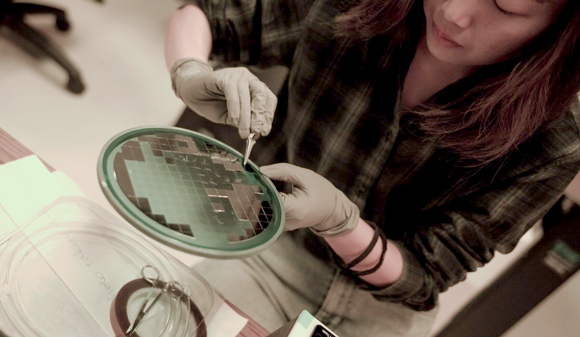
Researchers from California Institute of Technology (Caltech), Pasadena, California, USA, have developed an Additive Manufacturing fabrication technique to manufacture nanosized metal parts. The new technique is similar to work previously undertaken by the team, which produced micro-sized metal parts with features about as thick as three to four pieces of paper. At the nanoscale, these features are around a thousand times smaller.
In the new process, the team also discovered that the atomic arrangements within these objects are disordered. At a large scale, this disorder would render these materials weak and of low quality. However, in the case of nano metal objects, this atomic-level disorder has the opposite effect: these parts can be 3-5 X stronger than similarly sized structures with more orderly atomic arrangements.
The work was conducted in the lab of Julia R Greer, who is the Ruben F and Donna Mettler Professor of Materials Science, Mechanics, and Medical Engineering. She is also the Fletcher Jones Foundation Director of the Kavli Nanoscience Institute. The details of this work are described in a paper published in the journal Nano Letters.
The process is reported to begin by preparing a photosensitive ‘cocktail’ consisting mainly of a hydrogel, which is a type of polymer capable of absorbing water many times its own weight. This cocktail is then selectively solidified using a laser to create a 3D scaffold in the desired shape of the metal objects. In this study, the objects created were a collection of small pillars and nanolattices.
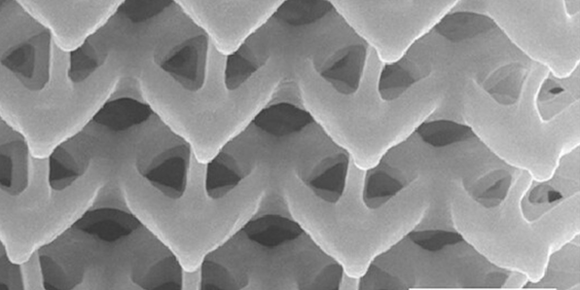
The hydrogel parts are infused with an aqueous solution containing nickel ions. After saturating the parts with metal ions, they are sintered until all the hydrogel is burned out. This leaves behind parts that are the same shape as the original, but have now shrunken and composed entirely of oxidised metal ions (bound to oxygen atoms). In the final step, the oxygen atoms are chemically removed from the parts, converting the metal oxide back into its metallic form.
In the last step, the parts develop their unexpected strength.
“There are all these thermal and kinetic processes occurring simultaneously during this process, and they lead to a very, very messy microstructure,” Greer shared. “You see defects like pores and irregularities in the atomic structure, which are typically considered to be strength-deteriorating defects. If you were to build something out of steel, say, an engine block, you would not want to see this type of microstructure because it would significantly weaken the material.”
However, Greer says that they found the exact opposite: The numerous defects that would weaken a metal part on a larger scale actually strengthen the nanoscale parts.
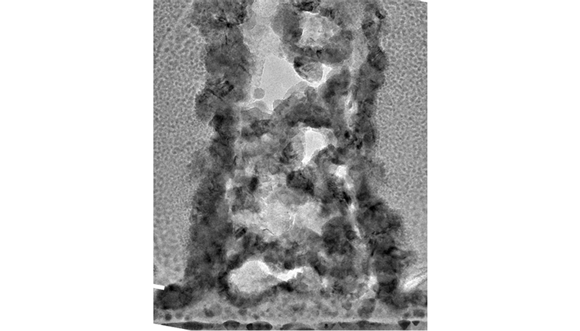
When a pillar is defect-free, failure occurs catastrophically along what is known as a grain boundary. This is the point where the microscopic crystals that make up the material butt up against each other. However, when the material contains numerous defects, a failure cannot easily propagate from one grain boundary to the next. As a result, the material will not suddenly fail because the deformation becomes distributed more evenly throughout it.
“Usually, the deformation carrier in metal nanopillars — that is, a dislocation or slip — propagates until it can escape at the outer surface,” stated Wenxin Zhang, lead author of the work and a graduate student in mechanical engineering. “But in the presence of interior pores, the propagation will quickly terminate at the surface of a pore instead of continuing all the way through the entire pillar. As a rule of thumb, it’s harder to nucleate a deformation carrier than to let it propagate, explaining why the present pillars may be stronger than their counterparts.”
Greer believes that this is one of the first demonstrations of Additive Manufacturing of metal structures at the nanoscale. She notes that the process could be used to create various useful components, such as catalysts for hydrogen, storage electrodes for carbon-free ammonia and other chemicals, and essential parts of devices like sensors, microrobots, and heat exchangers.
“We were originally worried,” she added. “We thought, ‘Oh my, this microstructure is never going to lead to anything good,’ but apparently, we did not have a reason to worry because it turns out it’s not even a detriment. It’s actually a feature.”
‘Suppressed Size Effect in Nanopillars with Hierarchical Microstructures Enabled by Nanoscale Additive Manufacturing’ is available here. The research was funded by the US Department of Energy.
Download Metal AM magazine
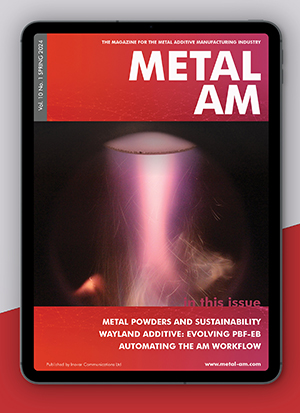