Flight critical control system optimised for NASA Orion spacecraft
April 11, 2022
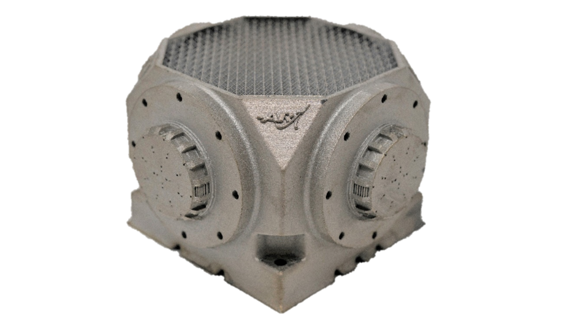
As already announced, NASA intends to place the unmanned Orion spacecraft into lunar orbit in 2022, followed by crewed moon landings, construction of lunar habitats and supporting infrastructure, and ultimately, prepare for a visit to Mars. According to NASA administrator Jim Bridenstine, the space agency and its partners will accomplish this by the end of this decade and do so with half the buying power it had back in 1964, when Apollo development was at its peak.
One of the key technologies that will enable this ambitious plan is the use of Additive Manufacturing. “As with any complex endeavour, the more affordable you can make it, the greater the chance that you will ensure its completion – and the moon is no different,” stated James Horton, aerospace engineer and mission architect at Aerojet Rocketdyne. “Metal AM plays a key role in achieving these goals.”
Aerojet Rocketdyne, Los Angeles, California, USA, will use metal Additive Manufacturing as an affordable alternative to traditional manufacturing to ensure the organisation gets more for its money.
For over two decades, Aerojet Rocketdyne has invested extensive time and resources into leveraging Additive Manufacturing, focusing most of its energies on Laser Beam Powder Bed Fusion (PBF-LB) technology. With this experience, the company has designed and integrated additively manufacture end-use components for a variety of projects, including the RS-25 engines which will carry NASA’s Artemis mission into space.
Horton has stated that metal Additive Manufacturing, used in conjunction with advanced design and simulation software, gives aerospace engineers “an entire buffet of solutions that were completely unavailable to their predecessors, providing the unparalleled ability to innovate without compromise.”
Optimised reaction control system
One example of this is a critical subsystem that Apollo engineers in the 1960s knew as a quad reaction control system (RCS). The Apollo RCS included four individual R-4D bipropellant thrusters that used hypergolic (spontaneously igniting) nitrogen tetroxide and hydrazine as propellants. Every lunar lander and service module had four quads, each of which generated more than 45 kg of thrust to control the spacecraft’s roll, pitch, and yaw during flight. Following a series of acquisitions, Aerojet Rocketdyne took ownership of the R4-D, intending to use the reaction control system on the future spacecraft.
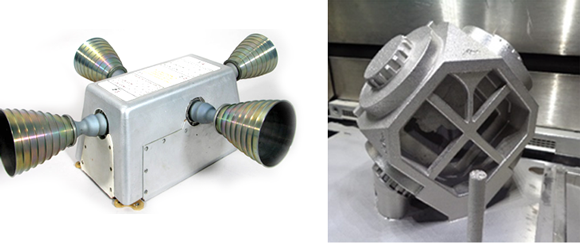
Given that the original RCS design is more than sixty years old, Horton and his team recognised an improvement opportunity and began what would become one of the many internal research and development (IRAD) projects intended to optimise the systems required for the Artemis programme and other commercial lunar projects. In the case of the RCS, the team looked for ways to reduce the number of engine parts, increase its reliability, and make assembly and serviceability easier, all of which would decrease project costs while enhancing spacecraft performance. Metal Additive Manufacturing is said to have checked all these boxes.
“During the entire Apollo programme, NASA produced more than 650 thrusters to support six Moon landings,” added Horton. “It was a huge number, which led us to believe we were onto something big – we knew that if we could bring affordability to the RCS, we could make a positive impact on any this and future programmes.”
They also wanted to take advantage of rocket fuel advancements: propulsion engineers had learned over the years that adding 25% nitric oxide to nitrogen tetroxide would reduce its freezing point from -9ºC to -55ºC. Since this reduces the mass and power consumption of the heating systems needed to prevent frozen fuel lines in space, it presented Aerojet Rocketdyne with a significant opportunity, with a large caveat: the resultant MON-25 fuel is unstable.
To overcome this issue, the team additively manufactured special injector geometries that could burn the fuel in a stable manner. A concept model was soon designed and built from the nickel-based superalloy Inconel 718, but unfortunately, ran into limitations with the available metal Additive Manufacturing machine.
“We had to build the RCS injector body at a 45º angle, due to overhang concerns, and also add in a series of large support structures to prevent thermal warping during the build process,” Horton stated. “The supports would need to be machined away afterward, adding cost to the product, while the orientation angle created less than desirable surface quality. There was definitely room for improvement.”
At the same time, the team realised there was room for substantial lightweighting and topology optimisation. Horton noted that they’d already eliminated some unnecessary material during the redesign, but it was far from perfect.
“Every single pound of material you can remove from a spacecraft saves money on launch costs,” he said. “That’s why we turned to nTopology for help with optimising the design, and then Velo3D to build it for us.”
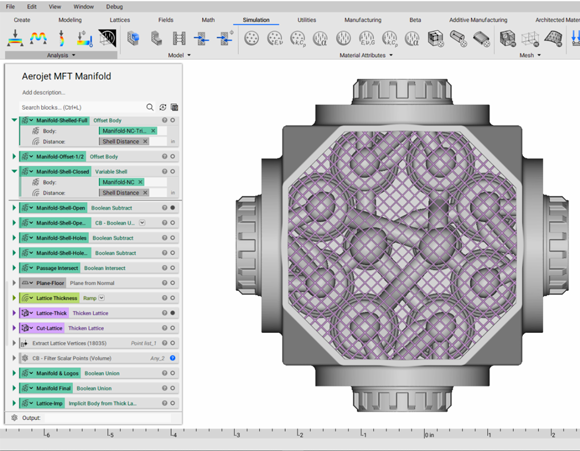
Technicians from New York-based engineering and design software company nTopology were consulted and were able to hollow the injector body’s blocky structure, leaving consistent wall thicknesses around the complex fluid ports and channels while eliminating stress concentration areas. They then filled the resultant void with a thin lattice structure, increasing its strength and stiffness while adding only minimal weight. From start to finish, nTopology was able to cut the quad injector’s mass in half.
The Aerojet Rocketdyne team sent the optimised part file to Velo3D’s. Here, the Aerojet team received further good news: using titanium instead of Inconel would further reduce the weight. and as Velo3D technical sales engineer Gene Miller noted, there’d be no need to build it at an angle or use the big, blocky supports as in the first iteration.
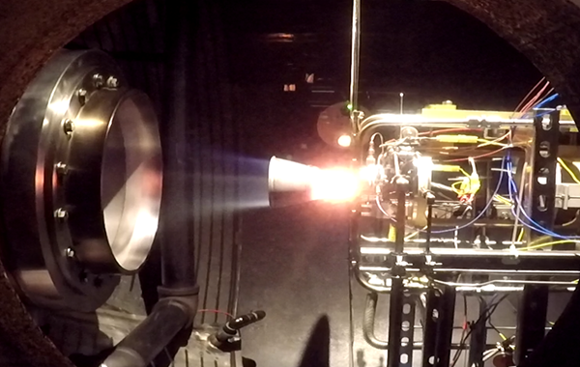
“Our proprietary, pre-print software is intuitive enough to recognize different geometric features and apply specific laser parameters to those areas so that they print as efficiently as possible and without the need for added support material,” stated Gene Miller, Technical Sales Engineer, Velo3D. “In addition, we’re one of the few metal AM system providers that can successfully print large complex titanium parts without cracking. We have a unique solution to mitigate accumulated internal stress within the printed material, and can avoid cracking more so than other printers on the market.”
The final result is an RCS thruster that is 1/5 the mass, 1/2 the size, and 1/3 the cost of a conventionally manufactured version. And since it contains far fewer components, it’s also easier to assemble, with less chance of failure during operation.
Horton concluded, “We’ve shown that by leveraging Additive Manufacturing and advanced software technology, we’re able to interject affordability, reduce lead times, and greatly improve upon system performance compared to the way we built parts in the past. Our next step is to demo this proof of concept, bringing it into actual field testing and, hopefully, final qualification. From there, it’s headed into space.”